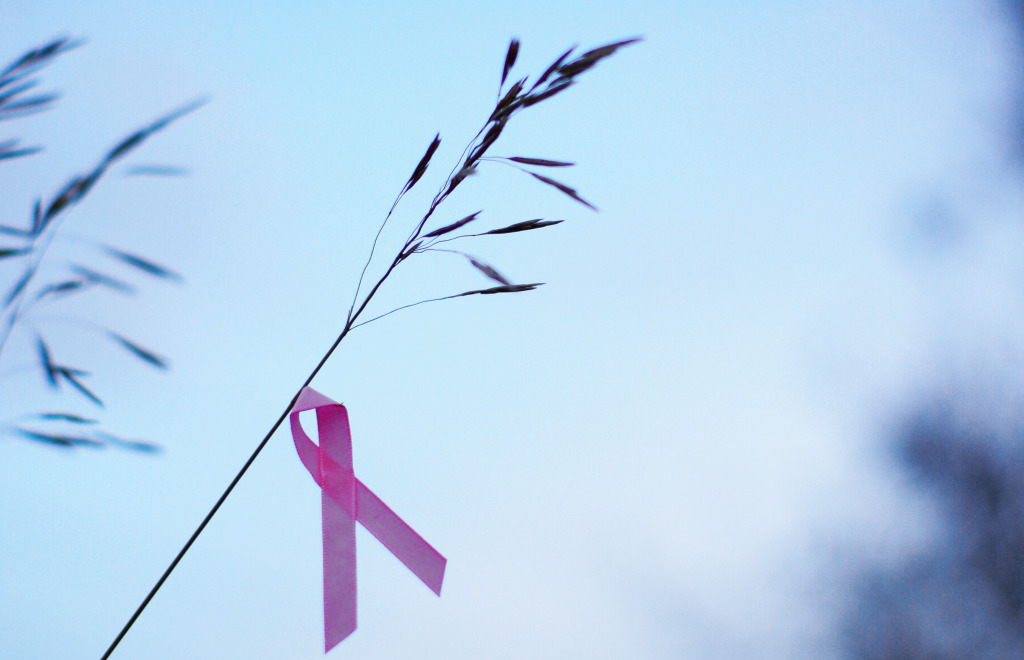
Can cancer research survive funding cuts, Congressional gridlock, and workforce attrition? Image: Paul Falardeau
Staring at Dima’s laptop screen, all I see are a couple lines of esoteric text supported by numerous brackets and parentheses, but a confident press of the enter button sends this indecipherable incantation to a supercomputer with the computational horsepower of 96 desktop workstations, which then busily hums away to the instructions dictated in the code. It’s hard to believe that this isn’t a scene out of some cyberthriller or even the relatively quotidian day at the Google campus in Mountain View. This is how Dima Gorenshteyn, a PhD student in quantitative and computational biology, is conducting cancer research at an outdoor table. Usually the tasks he sends to the computer can take days to compute, but he is kind enough to show me some of his preliminary results. “R is amazing,” he declares, fawning over his choice of statistical programming software, “there’s an elegance to the syntax that allows me to do almost anything.” As a fellow programmer, I can appreciate his sentiment: colorful plots summarizing complex data, like gene expression levels or ethereal statistical numbers, into figures immediately ready for publication in the increasingly competitive cancer literature, figures that would take me days to even conceive, let alone produce. Professional envy aside, it provides me with the stark realization that cancer research has dramatically transformed even within the four years since I started my own doctoral program. No longer are cancer biologists only seen toiling away at a lab bench, growing tissue cultures and mixing reagents. They can also be spotted furiously coding away at a computer screen, chatting about Bayesian learning algorithms as easily as they compare notes on different microscopy methods.
The tranquility of the autumn day belies the anxiety I feel gnawing away at me. Dima continues to punch out code on his laptop as the sun recedes behind ominous clouds. I pull my jacket tighter around me, unsure of where to look for the source of my growing unease. Against this high-tech background of cyberpunk landscapes, I can’t suppress the shudder as a chilling wind breaks its way through my muffler and penetrates my skin. I, like any other scientist, know that there’s a storm coming.
When Genes Meet Algorithms
Cancer research has seen quantum leaps over the past decade, and not only has its scope expanded, its depth is staggering. Performing a cursory search on PubMed, a clearinghouse of biomedical information run by the National Institutes of Health, more than 56,000 research articles have been published on breast cancer from the beginning of 2010 to the end of 2013, not including clinical case studies, scholarly literature reviews, or conference papers. Looking at the trend line, it seems that each year brings at least a thousand additional publications on top of the total number published the year prior, and this does not include searches for lung, colorectal, or other types of common cancers.
This explosive growth in cancer research does not at all shock Dr. Yibin Kang, a professor of molecular biology at Princeton University and a world-renowned expert in breast cancer metastasis. “The information content in cancer biology is going to be increasing exponentially in the next decade,” he states. The increase in information is also commensurate with the increased sophistication of biological and computational tools, and more importantly, the merging of the two. The rise of bioinformatics—the use of computational and statistical tools to manage biological information and mine thousands of experiments for insights—has accelerated biomedical research, especially cancer research, beyond what was previously conceivable. In the past, as Dr. Kang explains, many of the findings in cancer biology were serendipitous after years of work at the laboratory benches. Researchers would spend months growing cancer tissue cultures or cultivating genetically modified mice to probe the effects of a subset of genes on cell shape and composition or tumor progression. Now, with the availability of databases, such as the Cancer Genome Atlas, and new technical breakthroughs, like the ability to sequence the DNA of single cells, cancer research can be much more directed, allowing scientists to single out candidate genes from a list of over 30,000 catalogued in the human genome.
This ability to single out candidate genes and genetic pathways is crucial for understanding cancers and developing cures. The human body is an incredibly complex system of various biological molecules—broadly classified as proteins—that interact with one another at multiple levels of hierarchies. These proteins are encoded in DNA as sequences of molecular units called “nucleotides”, and the term “gene” is used to denote these regions, which include information on how to build proteins. However, it is not only protein structure that is encoded in these genes, but also the regulatory mechanisms that allow or prevent a protein from being constructed. To further complicate things, many proteins and even DNA sequences also interact with one another, creating intricate networks that affect everything from cell division to tissue generation. When these regulatory networks and pathways are damaged, the results could be disastrous for a potential patient, leading to various diseases and disorders, including cancers.
Cancers are especially complicated diseases, as they entail a number of malfunctioning pathways. In 2000, Douglas Hanahan and Robert Weinberg outlined six “Hallmarks of Cancer” that underlie most cancers: (1) cancer cells encourage their own growth; (2) they ignore biological signals that inhibit their growth; (3) they resist naturally programmed cell death; (4) they increase the number of blood vessels to supply nutrients to themselves; (5) they can multiply forever; and, (6) they are able to invade and colonize different tissues. Each of these hallmarks independently can entail hundreds of genes, meaning that the combined space of cancer-related genes can reach well over a thousand. To probe each of these genes would require an insurmountable amount of work, as cloning a single variant of these genes into model organisms can take months, if not years, and that does not even address the fact that cancers can be caused by a combination of defective genes, not just a single one. By collecting previous results into large databases and combing them through for correlations between gene variants and pathologies, bioinformatics has provided cancer researchers a valuable time-saving measure by pointing scientists towards the specific pathways that are implicated in tumor genesis and progression in an unbiased and high information content manner.
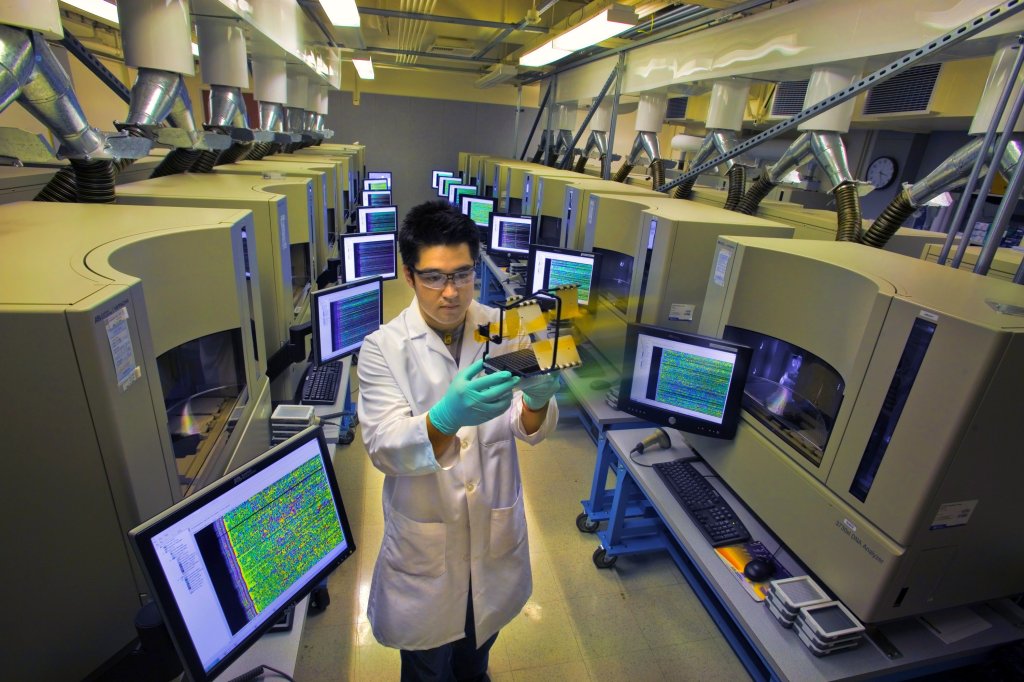
Breakthroughs in DNA technology and the application of computational frameworks have accelerated the pace of cancer research. Image: Berkeley Lab
The rise of bioinformatics, and more generally, “computational biology”, has slingshot Dr. Kang’s research by orders of magnitude, and he argues that the insights coming out of data mining could have taken upwards of ten years without the aid of these new hybrid frameworks.
“Here’s an example,” he explains breezily, “In the past, when you had an interesting phenotype or trait in the sample organism, you had to do all sorts of genetic backcrosses with reference samples to try to map out the genetic defect. Now what you do is simply take your samples, figure out its gene signatures using microarrays [a grid of tiny DNA sequences used to measure gene expression levels of thousands of genes simultaneously] or sequencing, and by looking at the output, you can pretty quickly isolate interesting pathways. And since you’re looking at the entire genome, instead of testing for a single gene, your hypothesis generation [the process of guessing which genetic pathways are most important in cancer development] is less biased and far more comprehensive. This is a sweeping change.”
The upswing in bioinformatics is also paired with the complementary breakthroughs in biotechnology. Take the example of whole genome sequencing—mapping out the nucleotide sequence of all DNA in an organism. The Human Genome Project, which took thirteen years to complete with a total operating cost of $3 billion, required the cutting down of the genome, some 3 billion nucleotides long, into fragments that were then sequenced in runs of about 2,000 nucleotides long. Today, the equivalent number of nucleotides can be sequenced within days using next-generation sequencing technologies, setting researchers back about an underwhelming $5,000 according to the National Human Genome Institute (although it’s worth noting that these cost estimates assume that a reference genome to be used as a template is available for the sample). These amazing gains in cost-efficiency mean that we can now study genetic and protein profiles at the single-cell level, allowing researchers to isolate subtle signals from individual cells that would otherwise be lost in statistical noise. This is a critical new ability in cancer research, as cancerous tumors are genetically heterogeneous, and each cell within the tumor can have a different genetic profile. Development of other genetic techniques has also made research far easier and more efficient, particularly in cloning. The GAL4/UAS system, first isolated in yeast, allows scientists to express various genes, including human genes, in specific tissues of model organisms like fruit flies and mice. Constructing transgenic mice strains in the past was an effort worthy of a doctoral dissertation, yet now a graduate student can simply order mouse strains from standard libraries and consortia instead of having to build her own mice from scratch.
This towering wave of research has also made its way into the clinical world, as new clinical tests that capitalize on the bioinformatics revolution are starting to become available for cancer patients. One of the earliest success stories is how we treat chronic myelogenious leukemia, or CML, a cancer of white blood cells. In the past, CML was essentially a death sentence, as it required bone marrow transplants for treatment. In 2001, the FDA approved Gleevec, a member of the class of drugs known as tyrosine-kinase inhibitors, which can target specific molecular profiles of CML cells, instead of killing all cells indiscriminately. “CML used to be a uniformly fatal disease,” says Dr. Ben Ho Park, a professor of oncology at Johns Hopkins School of Medicine and also a breast cancer specialist, “but now patients can live with the disease.” Dr. Park also highlights the development of drugs targeting the HER2/neu gene, the overexpression of which is implicated in 15 to 30% of breast cancers. “Now that we know a lot more about the genetics and the pathogenesis of breast cancers involving HER2,” he explains, “we can develop drugs that specifically target cancer cells only.”
The two examples above relied on research conducted in the 1980s and 1990s, and the discoveries since then are beginning to find their ways into novel clinical technologies. Companies like Foundation Medicine are working on platforms that take individual patients’ genetic data and compare them against giant databases of correlations between different types of cancers and drug efficacy. Such technologies would allow for the personalization of medicine, which not only means a more targeted and effective treatment regimen for patients, but also the possibility uncovering critical clinical associations between biological molecules involved in cancer and the drugs used to treat them. This could result in the discovery that a treatment used for one type of cancer might actually be also effective in fighting another. Technologies spurred by advances in bioinformatics and genetic research have also streamlined existing clinical screens. Traditional prenatal diagnosis for genetic disorders, such as Tay Sachs disease or Down syndrome, required the physician to surgically puncture the womb in order to gather fluid samples from the amniotic sac, the membrane that surrounds the fetus. Today, companies like Natera, Sequenom, and Ravgen are able to detect a battery of hereditary diseases by simply drawing blood from the mother. And that’s just the beginning.
“There is a lot of stuff out there that’s pretty new and we’re continuing to investigate treatment methodologies,” Dr. Park notes.
This explosion of new biomedical tests and treatment strategies all stem from basic and clinical research performed at universities and research hospitals funded mostly by federal agencies, most notably the National Institutes of Health. The complexity of the diseases studied has required an equally complex set of approaches, and never before has cancer research been so complex. Yet while the stakes are high, the future of biomedical research is rocking precariously out of balance. Scientists are panicking over a prolonged funding shortage. The research community, along with clinicians and patients, are faced with the anxiety of a crumbling academic infrastructure, and many are uncertain as to how much longer it can survive.
Crisis in the Academy
Given the rising prevalence of cancers in human populations and the revealed complexity of their biology and treatment, demand for resources has never been higher for cancer research. The publication of a single research article has an impenetrable, labyrinthine cost structure. Direct research costs can include chemical reagents, animal and tissue culture care, a battery of analytical pipelines like sequencing or biochemical assays, specialized equipment, and especially now, computation time on supercomputer clusters, along with any proprietary software licenses necessary for computational analysis, which can run into the thousands of dollars for a single volume license. The indirect costs are even further fragmented and variegated—maintenance of research facilities; tuition and salaries for graduate students, postdoctoral researchers, and research support staff; institutionally shared resources, such as high-powered microscopes, sequencing machines, and of course, supercomputers; academic journal and database subscriptions (a heated issue in itself); conference and travel grants; publication fees; and, perhaps increasing by most, safety and regulatory compliance costs. Each federal research grant thus breathes life into an entire economy of scientific activity.
A recent Science paper written by an international team of labor economists has tried to trace how researchers are using these federal research grants in an effort to understand the cost structure of scientific research and its effects on the national economy. By examining the spending patterns of nine large research universities in the Midwest, the authors report that the funds are used to employ five categories of scientific workers: faculty, graduate students, postdoctoral fellows, research staff, and undergraduate students, and the distribution of support depends on the specific agency. In aggregate, faculty researchers account for less than 20% supported by federal funding, research staff account for one third of the personnel, students (both graduate and undergrad) also account for one third, and postdoctoral fellows account for roughly one tenth, indicating that the scientific workforce dependent on institutions like the NIH and the NSF is incredibly heterogeneous. Delving into purchasing data, the authors also found that while research grants were used to purchase from well-known large firms, there were tens of thousands of niche high-tech vendors that provided numerous research goods and services to laboratories, meaning that federal research spending may play a significant role in stimulating demand and innovation from these small suppliers. With the increasing pace of research, as well as the tantalizing feeling of being close to a major breakthrough overhanging, researchers have been pressured to discover further measures to maximize their output. Yet despite the wealth of the growing number of resources available to them, scientists have found it progressively more difficult to afford these resources, as federal funding for science has been shrinking, even well before the recession.
Most biomedical scientists remember the 1990s fondly as a golden age of federal funding. Between 1990 and 2003, the NIH’s total appropriated budget increased by over 150%, adjusting for inflation. Save for 1995, when the Republican Revolution stormed into Congress, the annual growth rates of the NIH budget were well in the positive. However, 2004 was the start of the downturn and the NIH budget saw negative growth during the entirety of the second Bush administration. In 2009, the American Recovery and Reinvestment Act of 2009 (ARRA) injected some life into the NIH, but with the election of the 112th Congress in 2010, the NIH once again saw its budget plummet. These figures refer to the total funds allocated to the NIH, including administrative costs and the funding for operations and research at intramural NIH research campuses.
To see the effect on university and hospital researchers—called “extramural research” by the NIH—one good metric is the obligations for “Research Project Grants”, which constitute the largest portion of grants for which researchers like Drs. Kang and Park apply. A similar story arises in terms of the annual growth rates. While each RPG is allotted a different amount of funds, the overall decrease in the RPG budget has also meant a decrease in the number of RPGs available. By 2013, the NIH cut 3,604 research project grants compared to the high in 2004. Roughly, this would mean that the number of researchers unable to secure funding through the NIH, the largest source of federal research funding (not counting weapons technology development funding through the Department of Defense), can score somewhere in the thousands, a conservative estimate, considering the fact that many researchers can be funded through multiple NIH grants. Note that this paucity of funds does not affect only the Principal Investigator (PI), or head researcher, of a lab group; it also affects funding for postdoctoral researchers, lab technicians, and graduate students employed by the PI. The advances in bioinformatics and cancer genomics compare starkly against the funding environment, and scientists are beginning to crack under the strain.
“If you compare now to 2000, I would say there is a big change in terms of the outlook of scientists,” says Dr. Kang. “When I was graduating from my PhD program in 2000, I was wondering whether to go to industry or academia, and my family and colleagues were very optimistic about a career in academic research. At that time, the funding rate was doubling, so if you had a good proposal, you had pretty much an almost guaranteed chance after a couple of tries to get supported. And now, over the past ten years, it has been slowly climbing down, sometimes even into the single digits, and at that level, there’s no guarantee of successfully funding good science. It’s becoming closer and closer to randomness.”
Francis Collins, the director of the NIH, and Sally Rockey, the NIH’s Deputy Director for Extramural Research, have also sounded the alarm, publishing a grim message on Dr. Rockey’s blog in September of last year. They note that with the sequester, a grant application has less than a one in six chance of being successfully funded, and furthermore, one quarter of the 650 grants being cut by the sequester are renewal applications, meaning that even current research is being halted in the hostile funding environment. They quote a nationwide survey by the American Society for Clinical Oncology, in which “28% of cancer researchers have decided to participate in fewer federal-funded clinical trials; 27% have postponed the launch of a clinical trial; and 23% have had to limit patient enrollment on a clinical trial.” In addition, they write, “If sequestration continues for a full ten years, the outlook for the U.S. biomedical research enterprise turns downright grim.
This is incredibly alarming to Dr. Kang, who has noted that scientific practice has already suffered. “The task of a scientist has now become to write lots of grants in order to try to get lucky. That’s really counterproductive, because you don’t want to spend lots of time writing grants. You want to spend time doing science, working with your students, and thinking about ideas and interacting with other scientists. But that’s the reality now; people are focused on finding ways to survive in science.”
Not only is the total amount of funds shrinking, the scope of research being funded is also becoming narrower. Jane (who asked to us to withhold her real name) is a postdoctoral fellow in the New York area looking to enter the academic job market, and she has felt firsthand the effects of the squeeze. “Funding priorities have limited the types of openings, as different departments are looking for new faculty in different ‘hit fields’ in which it will be easier to find funding. This affects the way I do science. I’m personally interested in some more basic questions, like how cells divide, but I have to stretch to find ways to see how my research interests address human health. While this has been helpful in providing a broader scientific context for my work, it has also limited my research plan that I’ve submitted as part of my job applications, which is essentially what I’m going to study over the next five years.”
Jane’s fears are well justified when looking at the distribution of NIH budget authority between “basic” (defined by the NSF as research “to gain more complete knowledge or understanding of the fundamental aspects of phenomena and of observable facts, without specific applications towards processes or products in mind”) and “applied” (“to gain knowledge or understanding necessary for determining the means by which a recognized need may be met”) research over the years. In 2002, basic research accounted for 58.8% of the budget authority, whereas in 2013, it tumbled down to 54%.
An even more threatening prospect is the possibility of congressional oversight of the grant review process. Over this past summer, there have been no less than four public showdowns between the NSF and Republican aides to Representative Lamar Smith (R-TX), chair of the House Committee on Science, Space, and Technology. In May of this year, Smith pushed forward the approval of the Frontiers in Innovation, Research, Science, and Technology (FIRST) Act, which would require the NSF to prioritize research that Congress deems to be “in the national interest.” Jane finds these developments incredibly unsettling, as she is skeptical as to how politicians like Smith, whose training is in law, can judge complex grant applications reviewed by top researchers in their respective fields without any significant scientific knowledge or training. “It’s very frightening to a scientist, particularly for someone like me who works on a model organism, to say that some point, someone who is not a scientist will pick up my research and say, ‘Hey! This person is working in a bacteria or a worm or a mouse, which is not human, not a human cell, so I don’t understand why we should give them money,’” she shudders.
Furthermore, basic research is what seeds the new technologies and treatment outcomes, Jane and other scientists interviewed for the article have repeated. Without the discovery of the GAL4/UAS system in yeast, we would not have the model mouse libraries powering cancer research; without probing plant biology, we would not have discovered the phenomenon of RNA interference, a staple genetic tool used in modulating gene expression; without experimenting on bacteria, we would not have penicillin or any antibiotics. These technologies have depended on serendipity, stemming from “unknown unknowns,” to borrow the phrase from Donald Rumsfeld. Limiting research scope based on national interest, scientists argue, limits discoveries and can have incredibly adverse consequences.
“One thing that Ebola makes crystal clear,” Jane explains, “is that a laser focus of the government on the diseases that will affect the American people or the things that are nationally appropriate is going to come back to bite you on the ass when a disease that we have not prioritized has come onto our shores.
On top of the public health concerns, the funding crisis has dire downstream economic concerns as well. Dr. Manish Gala, a junior faculty member in gastroenterology at Massachusetts General Hospital, notes that the commercialization of technologies stemming from research, especially in biomedicine, has been at the hands of the very academics who have conducted the research. “Liquid diagnostics, like what you see at Natera, have all been started by professors,” he says. Technology transfer, the patenting of inventions as a result of research from federal funds, has flourished after the Bayh-Dole Act of 1980, and the entire industry of commercial biotechnology, including large portions of agriculture, owes its success to the greater ease of commercializing scientific research. “You get your money’s worth on public spending on federal research,” summarizes Dr. Gala, who also co-founded a genomic medicine startup.
More immediately, there are clear short-term economic benefits produced by academic science. As the aforementioned Science paper presents, these federal research funds are used to employ a large, diverse scientific workforce, as well as stimulate demand for products and services from tens of thousands of high-tech companies. While the study was a preliminary pass through the data obtained, the authors write, “If, as our analysis suggests, the process of scientific research supports organizations and jobs in many of the high skill sectors of our economy, rapid changes in funding could have substantial downstream consequences.”
All of these concerns resonate deeply with Dr. Eric S. Lander, a leading genomicist, director of the Broad Institute in Cambridge, MA, and one of the leaders of the Human Genome Project. In an interview with James Fallows of The Atlantic, he argues that the current funding climate for biomedical research is deeply disturbing for the future of bringing the benefits of cancer genomics to the public. His main message is that the risk-averse funding environment is ultimately delaying possible cures for cancer by generations.
“[I]f you aren’t prepared to waste money on things that might not work, you can’t possibly do things that are transformative,” he says, “[b]ecause for every successful transformative idea, there’s five times as many nonsuccessful transformative ideas [sic]. Nobody knows how to figure out in advance which ones they’re going to be.”
He further hits on one of the looming concerns regarding the future of scientific research: “We’ve got an amazing cadre of young people coming into the field, and they have this cognitive dissonance right now. On the one hand they see unbelievable opportunities, and on the other hand, for the first time they see the nation decreasing funding for biomedical research.”
And for young scientists, particularly postdoctoral researchers, the future is incredibly bleak.
The Invisible Scientists
Postdoctoral researchers, one of the most overlooked population segments in the national debate regarding scientific funding, serve as a perfect lens into the future of America’s scientific workforce. In the past, researchers who have been conferred their doctoral degrees would then join academic institutions as junior faculty members and begin their independent research careers. Postdoctoral fellows were considered to be temporary apprenticeship positions to further their scientific training under the mentorship of an experienced faculty member. What had initially started as a transitional two to three years has now bloated to encompass six to seven years of academic purgatory, the equivalent length of a PhD program, particularly in the biomedical sciences. This means that postdocs are stuck in a “holding tank” for nearly another decade and can reach age forty before they receive their first significant federal research grant. During this entire period, postdoc pay is set by the NIH at $42,000 in the first year and climbing to only $55,272 for seventh-year postdocs. Tara (who also asked to withhold her real name) knows this all too well as the chair of her university’s postdoctoral association.
“It’s all very stressful,” she says, laughing nervously. “You’re in your thirties with very little financial stability. I didn’t think that I’d be so uncertain about my future; I thought by now I would have set down roots, maybe even start a family, but it’s tough. There’s a fundamental existential stress behind all of this. I constantly ask myself, ‘What am I doing?’ and it doesn’t help that there are few community support structures for postdocs out there. I love doing science, discovering something that nobody knew before, and you have to find the internal motivation to continue. You have to love what you’re doing; otherwise you’ll have a mental breakdown.”
This lack of support structure is a common complaint found in the postdoc community. PhD Comics, a widely read webcomic depicting the lives of young scientists, has devoted an entire story arc to postdoctoral fellows, illustrating them as “ghosts” on university campuses. This portrayal of postdocs is not far from the truth, as it wasn’t even until 2007 that the NIH and NSF have adopted an official definition of a “postdoc.” The NIH has admitted that it does not know how many postdocs are working in research institutions, and in their 2012 report by the Biomedical Research Workforce Working Group, they call for better tracking of postdoc outcomes. This comes as no surprise to Ashley (real name redacted), who has found it difficult to navigate as an invisible postdoc in the biological sciences.
“Universities can’t even agree on an administrative level what being a postdoc means,” she claims. “At some places, postdocs are faculty and get faculty-level benefits. Other places they’re staff. Some places even count them as special types of students. At my institution, we’re none of the above. Our ID cards say ‘staff’, but we don’t get all the staff benefits, and even when I was jointly paid through my boss’s research grant and the university, I couldn’t get staff housing benefits. And now that I have external funding, I’m considered ‘external’, even though the university still takes a large portion of my funding as overhead. According to our postdoc association, the university doesn’t even keep a list of postdocs, which further isolates and marginalizes us.”
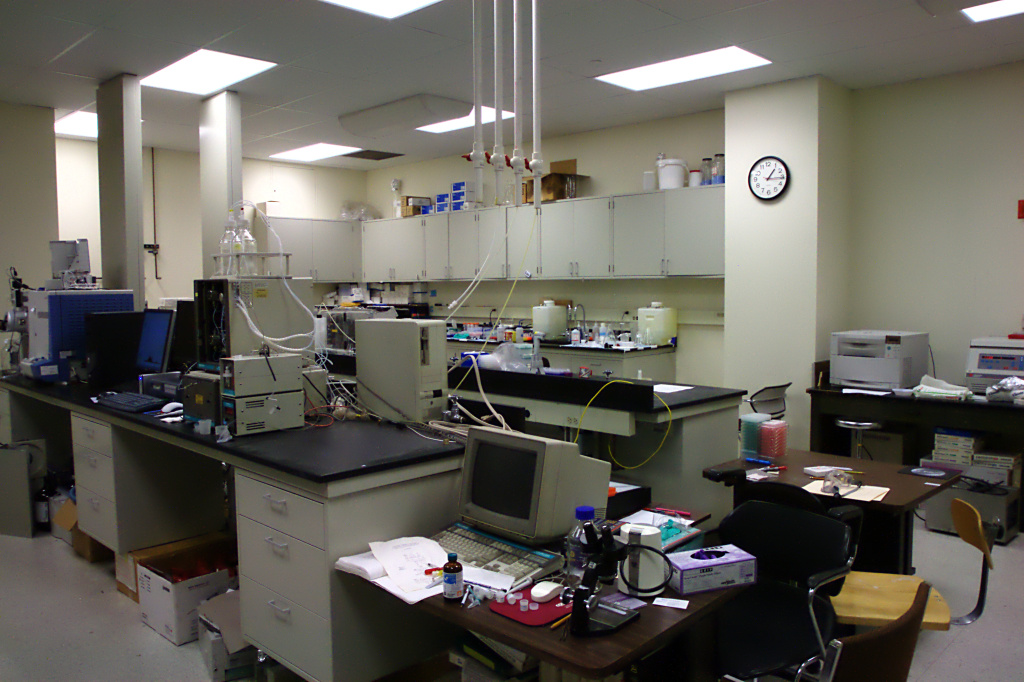
Postdoctoral researchers: the forgotten ghosts left behind in the national discussion on science funding. Image: Michael Pereckas
The postdoc crisis is compounded by the fact that more and more doctoral degrees are being awarded each year. One widely circulated Nature Biotechnology figure summarizes the state of the academic job market simply by comparing the number of PhDs awarded against the number of new faculty positions open annually in the sciences and engineering. In 2011, more than 35,000 scientists were conferred a PhD, while little more than 2,500 academic positions accepted applications. That means for those who graduated in that year alone, there were university appointments for less than one in seventy. This does not include the even greater number of postdocs who have graduated since 2004, if we take the seven-year postdoc length to its extreme. It’s important to note that these data are not limited to PhD earners in the biomedical sciences, but as Slate’s Jordan Weissmann reports, the abysmal career outcomes for young scientists and engineers are seen across all disciplines and research fields. With dwindling numbers of available positions and funding becoming even tighter, the working dynamic between hopeless postdocs and their struggling PIs may also be deteriorating.
The NIH has noted this concern in its workforce report. In a qualitative survey they sent out to biomedical researchers, “Supply and Demand” of scientific labor was found to be one of the top concerns, and they explicitly stated that many researchers commented that the current structure of the research workforce resembled a “pyramid scheme”: PIs would employ graduate students and postdocs as cheap labor, as opposed to hiring mid-level staff scientists. Ashley sees this as confirming her assessment that the research infrastructure is stacked against postdocs. “As far as I’m concerned, my PI has all the power in the world, because the one thing you need to get out of your postdoc experience is your recommendation letter from your boss,” she says, noting that the future success of a young scientist is highly dependent on the quality of personal references. “What’s worse is that by your sixth or seventh year, they don’t want you to leave. You have all of this specialized knowledge, and for all you know, you can end up becoming a direct competitor to your PI. They don’t want you to go because you’re good and they don’t want you to go because you are the competition. For some bosses, it can be a very hard line to walk and be supportive of you.”
The situation is further complicated by the fact that most postdoctoral researchers are in their thirties. Jane, Tara, and Ashley have all highlighted this as a particular concern for women, as decisions about children come to the forefront. Tara notes that there are a number of postdocs with children in her association, and that’s a difficult balancing act, given the long hours and the low pay. Ashley mentions that hiring decisions can also be affected depending on the age of potential women hires, because there are few policies regarding university support for maternity leave: “It very well could be that your boss has to fund you for five months away from the lab bench from their research grants. That’s very scary for them and it’s understandable.” She reports that she has heard stories of hiring discussions taking into account the likelihood of bearing children. “All of these things are on the minds of postdocs,” she continues. “So here we are, trying to lead adult lives in our thirties, and yet, it’s like we’re reiterating undergraduate life, and we’re continuing to extend this period of postdoc limbo. It’s very rough.”
One of Tara’s concerns is that this extended strain on postdocs is leading to scientific burnout. She’s afraid that many are dropping out and becoming bitter about science. “I joined my postdoc lab a couple of years ago, wanting to start a lab of my own. Now, knowing the numbers as they are, I don’t know. If I get a really good paper, then I have a chance. If I don’t have the luck, I don’t know if I should even think about it.”
Jane adds, “It’s such a shame that such brilliant people, such great scientific minds are leaving science, not because they want to, but because they have to.
This issue is tormenting Jane daily, as this is her second year looking for an academic research position. She mentions that there is a great paucity in terms of the number of openings posted, and each position requires a customized research plan that fits within the particular institution. She is writing an application a day, because all of the requirements and deadlines are different. “It’s a little scary,” she confides in me, “I think it’s going to be a tough adjustment in the years ahead. I’ve looked into alternative careers in things like management consulting, and they’re not really anything I wanted to do. I don’t get excited by them. I always wanted to educate students and continue pursuing scientific research, and I’m going into this job search with the possibility that I won’t have a job when I come out of it.”
I ask her how she stays motivated in face of all of these dire statistics. She replies, “I’m 100% dedicated to getting the job. And once I get the job, I am 100% dedicated to getting tenure. I think I’ve already passed the phase in which I remained idealistic and say, ‘No, if I write a really great grant and say clearly what I want to research, then I’m going to be able to do it.’ I jumped that hurdle as a postdoc as I don’t believe that’s true. I’m focusing on maintaining enthusiasm for what I know I can secure funding. And what it really comes down to is this: I think if you really love science, you can be excited about many scientific questions. It’s easy to maintain that day to day enthusiasm that way.”
Both Tara and Ashley share her unbridled love for science. Tara considers it paramount to why she is sticking with her postdoctoral appointment for another three years, and Ashley is willing to do any type of science if she can be persuaded by how interesting the project is. But for each Jane, Tara, or Ashley, there are an untold number of postdocs who throw in the towel.
“How many people would be able to keep that internal drive as you do?” I ask Jane, who has friends and coworkers on both sides of the job search. “Amongst your colleagues, what’s the number that decide to stay?”
She sighs deeply, not wanting to give the grim answer. “Smaller and smaller every year. Fewer and fewer people every year. I feel like there are people I expected to go out and run their own labs who are not in science anymore. And it’s a little shocking. It continues to surprise me. An amazing number of people I have known decided to go work at granting agencies, management consulting, finance, pharmaceutical companies, and the administration side of science. Even people who I thought were fantastic scientists, and it even makes you second-guess yourself. You think, ‘if this person thought that they couldn’t make it in science, then what am I doing?’ And the problem is that’s not their decision. For me, this is my last chance on the job market. If I don’t get a job at the end of this academic year, I will go do something else. And that won’t be my decision. That will be a decision that was taken away from me.”
She then glances over the NIH budget graphs I had provided her.
“Wow,” she says, wincing. “That’s unpleasant.” She pauses, taking in the steep funding decline of the past decade, zooming in onto the nosedive during the sequester.
“It’s so . . . random,” she manages. “If you were unlucky and had to write a grant for that funding period, you just got screwed. Completely out of your control. And that’s so . . .”
It’s another moment before she can find her voice.
“There are those people who are willing to stick with it, emotionally or psychologically, or what have you. But even those people who are willing to do so sometimes don’t have the option to keep chugging along at a postdoc until a job comes around. If you can only get a job with a Nature or a Science paper, and you didn’t get your Nature or Science paper, even though you spent a lot of time trying to figure out whether you’d be able to come up with one before you go out to the job market or not . . . and for a lot of people, there is that concern too. There is the realization that there’s no Science or Nature paper in my future, therefore I might as well not try to apply for academic positions. I don’t even think that all those people who have left have left necessarily by their own choice.”
I can hear the exhaustion in her voice. Four years in college, five years in graduate school, six years as a postdoc. Fifteen years in training. Fifteen years of putting off real life. Fifteen years, all for this one last shot.
“Like I said, if I don’t get a job this year, if I don’t stay in academia, then it won’t be my choice . . .”
We share another moment of silence before either of us has the courage to continue with the interview.
“It’s hard.”
The Gathering Storm
The precipitous drop in federal funding for biomedical research has been a dominant narrative for the past decade in academia, and while there may be a lag in visible consequences for the broader public, the deleterious effects are beginning to appear. It can be seen in the number of clinical oncologists who are less willing to partake in federally funded clinical trials. It can be seen in the number of discontinued grants that no longer fund critical research in human diseases and basic science. Most alarmingly, it can be seen in the coming wave of young scientists leaving scientific research altogether, either because they are jumping off a sinking ship, or because they are being flung out by a monster storm raging beyond their control. These losses aren’t theoretical. These losses are occurring today, and the tempest is only getting more violent.
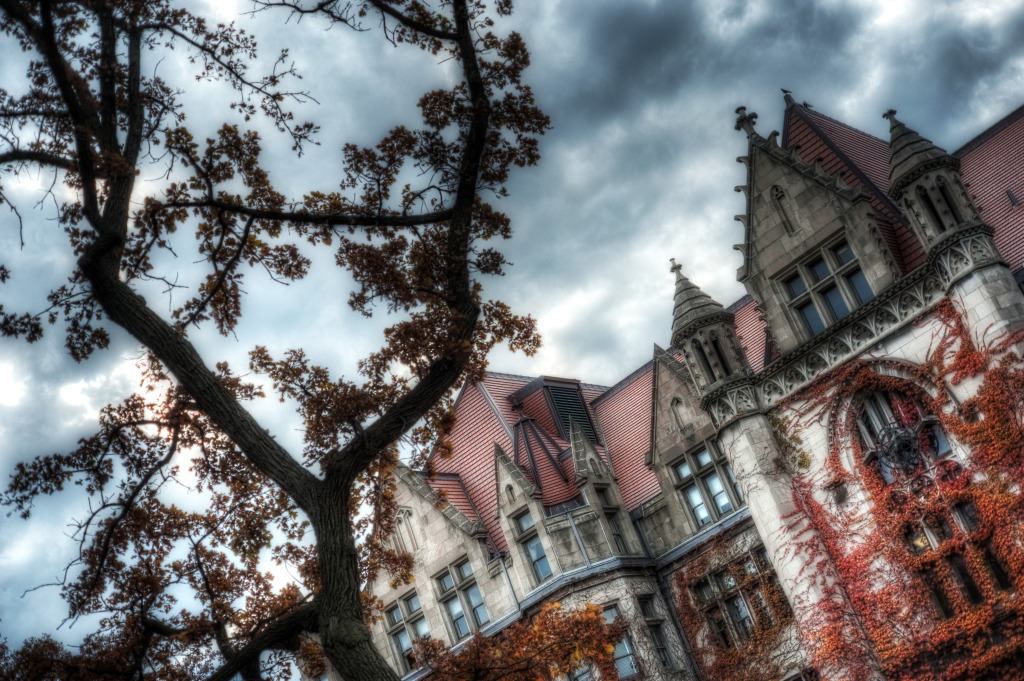
Dark days lie ahead for scientific research. Image: Justin Kern
One week before the publication of this article, I was contacted by a postdoc at a large medical school with crushing news. His PI had lost most, if not all, of his funding, and announced that the lab will be downsizing considerably. My contact had just finished his second year of postdoctoral research, and as it was a new project from the ground-level up, there is not enough time to salvage his work and prepare a publication within the months he has left. Having received his termination notice, he is paralyzed by the choice between taking the risk on another postdoctoral position or leaving academia altogether. These stories are becoming more common, and they will only increase if nothing is done about the funding situation in biomedical and the myriad of other critical sciences.
“What people have to understand is that graduate students and postdocs are funded by federal funds,” Jane points out. “They are paid via training grants from the NIH or from their advisors’ research grants from the NIH or the NSF, and that means that the American citizens have sunk a large cost into my education. I’m not really providing them with an investment return if I turn around and go into management consulting. They have set aside all of these funds to train people into scientists, and they’re not going to actually use these trained people, and that’s a lot of wasted money for the taxpayer. You can argue that we could be training fewer people, training fewer scientists, but I represent a large sunk cost for the American public. I should be used for what I’m trained to do.”
Ashley is visibly agitated. “I was always told the myth as a child that we need scientists, and I got here and I find out we don’t need scientists. There’s no money to do science. I’m going to have to fight so much to get money to do science that I won’t have any time do science.”
Researchers have grown increasingly desperate. Unable to rely on an intransigent Congress, scientists have had to start looking elsewhere for funding opportunities, including private foundations.
“I myself have received a lot of private grants,” Dr. Park mentions gratefully, “and it’s incredibly inspiring to see the public step up, because it shows how importantly the American public holds biomedical research as a priority. However, these grants are for smaller amounts. A typical NIH grant is about $250,000 per year over a period of 5 years, while a foundation grant would probably range around $100,000 annually over three years.” He also notes that he is particularly lucky that breast cancer has a large public awareness, whereas other diseases lack the attention he has been able to harness to his benefit. “These stop-gap measures have been helpful, but they’re not a cure-all or a panacea. They can’t replace federal funding. The public has definitely stepped up, but let’s remember here that the federal government is elected to represent the public. We’re really at a cusp right now in cancer research in making large improvements in clinical treatment, and the rug is being pulled out from under us.”
Dr. Kang has also sought alternative sources of funding and his lab is currently supported by funds from the State of New Jersey. He is able to draw from the New Jersey Commission on Cancer Research, and he has had state legislators, including the President of the New Jersey State Assembly, visit his laboratory in Princeton. “It was a surprisingly fulfilling experience,” he remarks. “People are actually willing to listen and everyone agrees that it’s an important thing to do. We as scientists really need to engage with society, engage with politicians.”
At the same time, he notes that state funds cannot substitute federal grants. “This is a very small appropriation, about $1 million. It’s usually in the budget, but every year we have to fight to keep it. What we need is a consistent, sustainable national policy. It can’t just be a one-time influx of money—all of these ups and downs are damaging to the research community.”
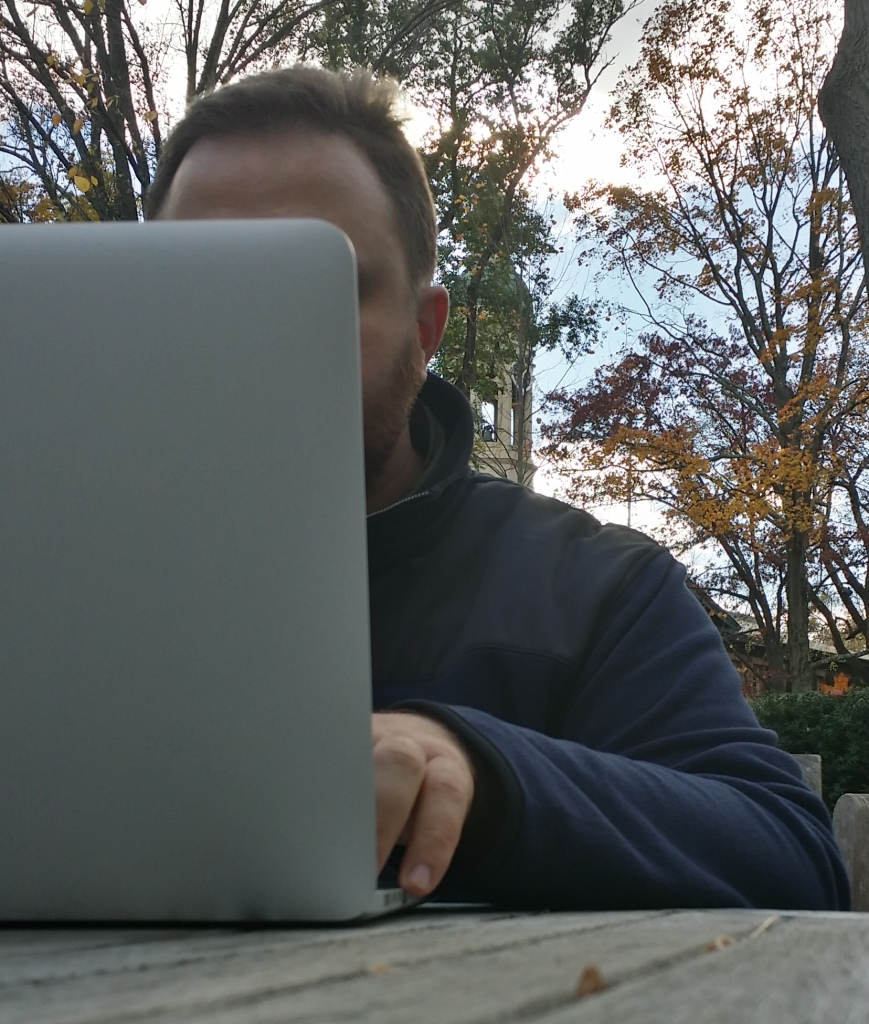
Dima Gorenshteyn, PhD student in quantitative and computational biology, is but one of the many young scientists who fear that they may have to leave science. Image courtesy of Dima Gorenshteyn.
The NIH has also responded to the crisis by trying to restructure what they can in the pipeline for young scientists. The recommendations in the 2012 Biomedical Research Workforce Working Group report include a number of changes for graduate students and postdocs, ranging from salary and fellowship increases to training period caps. They are also working on finding ways to diversify career options for graduate students and postdoctoral fellows. Many of these changes have been realized, but with the prospects of even further budget cuts, there is no way to predict their effects on career outcomes for young scientists.
None of this seems to impress Dima as he looks towards wrapping up his PhD. I ask him whether he is optimistic about his future as a cancer genomicist.
“Oh no,” he laughs hollowly. “I came in thinking that the nobility of science, the search of knowledge for its own sake, would be worth the sacrifices I would have to make along the way. I no longer think this is true, from what I’ve seen. I don’t think this current climate is good for me as a cancer researcher. The incentives, the internal desire to do something greater than the opportunity costs of financial and career security, the opportunity to try, whether or not you succeed—that’s why I came into science. But I don’t think this really exists anymore. It’s all about grant writing. It’s all about funding. I won’t be thinking about interesting questions, and I think this is how the funding crisis affects science. It’s killing it.”
Tara is a bit more optimistic about the long-term. “While I was in grad school, there grew this whole narrative that non-academic careers, which were previously taboo, were becoming okay. All of the graphs you showed me? That is why. And that’s still okay. Yes, funding is bad, but having educated scientists in the broader workforce—isn’t that good? Especially in policy and government, which will mean more research funding in the future. It’s a matter of how you frame the situation. This means that scientists should also better engage with the public and educate; otherwise we’re stuck with things like the Big Bang Theory, which is hardly an accurate representation of who we are and what we do. It’s going to be slow, though. I think it’s a generational change.”
Ashley is ready for a fight. “This is desperate. We can’t keep putting on all of these stopgap measures. We have to say, ‘This is it. We have to get serious. The time for a nuclear option is here.’ We can keep surveying people and talking about it, but we’re talking about the same things we were talking about when I started my PhD in 2005, and nine years later, no nuclear option has occurred. We keep saying, ‘Oh, it’s gonna get so bad that something’s gotta change.’ Well, nothing has changed. We’re here. Let’s do it.”
References and Further Reading
The Economist profiles the Cancer Genome Atlas
Researchers map out how cancer genomics can address unmet clinical needs
“Translational genomics” and the prospects for personalized medicine
Joseph V. Kennedy lays out how science funding is used in the United States in The New Atlantis
The National Science Foundation publishes an annual study of key economic data in science and engineering
Two articles, one in The Atlantic, the other in The New Republic, explore the consequences of the government sequester on scientific research
Federal funding agencies across the board face flat prospects, including but not singularly the NIH
William J. Broad profiles private sources of scientific funding in The New York Times
Bernhard Warner at Bloomberg Businessweek warns that private funding cannot replace federal research programs
Physicist and Nobel Laureate Steven Weinberg narrates the collapse of “big science” in The New York Review of Books, setting the scene as to how biomedical sciences can crumble as physics has
The Boston Globe profiles the postdoc crisis in the Boston-Cambridge area
Four prominent senior scientists, including the former president of Princeton University, call out for a major structural change in scientific work culture and training in The Proceedings of the National Academies of Science (PNAS)
The National Postdoctoral Association has published a number of white papers and reports on the postdoctoral experience
The Chronicle of Higher Education reports how graduate students are also victims of the current academic infrastructure
•
Daniel M. Choi is an Editorial Assistant at The Brooklyn Quarterly and a graduate student at Princeton University. His research focuses on quantitative methods of describing animal behavior. His professional interests also include science and technical communication, higher education, and interdisciplinary pedagogy. You can follow him on Twitter @dmh_choi.